The Apollo missions are most celebrated for putting human footprints on the moon, but their biggest contribution to science was the collection of rocks the astronauts brought home with them. To call these 382 kilograms of stone and regolith (the thick layer of crushed rock and dust that covers the surface of the moon and other planetary bodies) a treasure trove does not do them justice. Studying these samples in laboratories on Earth helped to establish the modern field of planetary science and gave us crucial insights into geologic processes that operate on all planetary bodies.
I was born too late to witness Apollo 11, but my life and career as a planetary scientist have been directly shaped by the samples brought back by the six missions that landed on the moon. For instance, some of my research concerns explosive volcanic deposits on the lunar surface. The data that I have used come from samples that were scooped directly off the surface by astronauts during Apollo 15 and 17. Other data were gathered by orbiting spacecraft that scientists built and sent to the moon as a direct result of the scientific and technical knowledge gained through the Apollo missions.
On supporting science journalism
If you're enjoying this article, consider supporting our award-winning journalism by subscribing. By purchasing a subscription you are helping to ensure the future of impactful stories about the discoveries and ideas shaping our world today.
In the past 50 years NASA has received 3,190 unique lunar sample requests from more than 500 scientists in more than 15 countries, according to Ryan Zeigler, NASA’s Apollo sample curator. Over the decades, he says, the agency has distributed more than 50,000 unique lunar samples, and currently 145 scientists are studying more than 8,000 samples in diverse fields, including astronomy, biology, chemistry, engineering, materials science, medicine and geology. Above all, the moon rocks have revolutionized our understanding of three major subjects: the nature of the lunar surface, the origin of the moon and the evolution of our solar system.
Ancient surface
Before we sent spacecraft and humans to the moon, our knowledge of Earth’s natural satellite was largely speculative, limited to observations that could be made from Earth.
These studies had suggested that the surface of the moon is extremely old because it is saturated with impact craters that must have taken billions of years to accumulate. When we finally landed on the moon, we knew for sure. After lunar rocks arrived on Earth, geochemists analyzed them for isotopes that decay over well-understood timescales and found that the moon samples were far older than most terrestrial rocks—between three billion and 4.5 billion years old.
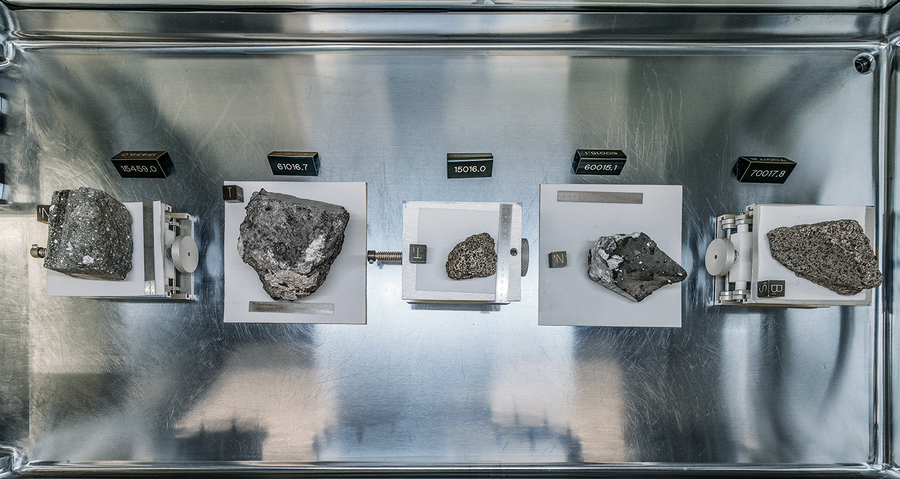
Five samples collected during the Apollo 15, 16 and 17 missions. Credit: Chris Gunn
Planetary scientists then made a connection that would affect virtually all subsequent studies of the moon and the other planetary bodies: they compared the first measured ages of lunar samples from the Apollo 11 landing site with the number of impact craters in the region where each was collected. Then they used this information to develop a model for how quickly impact craters form on the surface of the moon. Through this model, the Apollo sample sites serve as a kind of Rosetta stone, enabling scientists to estimate the age of any location on the moon (and even other planetary bodies) without visiting them.
At about 4.5 billion years old, the oldest sample is essentially the same age as the moon itself. Most rocks on Earth are much younger than four billion years because of the constant recycling of the crust by plate tectonics—a process that does not occur on the moon. Thus, lunar samples provide an important glimpse of ancient rocks from the early days of the solar system. They could even tell us about the young Earth. This March, researchers analyzing an Apollo 14 breccia (a rock type composed of other rock fragments welded together) determined that one of those pieces might not be a moon rock at all. Instead it may represent the first terrestrial meteorite—a rock that was ejected from Earth four billion years ago and then landed on the moon. After billions of years astronaut Alan Shepard picked it up and brought it home.
At the Apollo Sample Vault at NASA’s Johnson Space Center in Houston, technician Charis Hall Krysher handles the “Genesis Rock” (Sample Number 15415), a 4-billion-year-old old sample collected by the Apollo 15 crew on the lunar surface. This rock proved critical for helping scientists determine the origin and age of the moon. Credit: Chris Gunn
Lunar origins
Before Apollo, scientists had several competing ideas for how the moon and other planetary satellites formed. Perhaps Earth captured another body that passed too close. Maybe in its early days our planet spun so fast that a blob separated from the main body. Or maybe Earth and the moon may have formed at the same time from the original “protoplanetary disk” that gave rise to all the planets in our solar system. After the Apollo missions, however, we gained an entirely different picture.
Today the favored theory of the moon’s origin is called the giant impact hypothesis. This idea, based on evidence collected during the Apollo program, is that some 4.5 billion years ago, a body about the size of Mars (referred to as Theia) hit Earth, fragmenting itself and ejecting part of Earth’s crust and mantle into space. Eventually the ejected terrestrial material mixed with the remnants of Theia, accumulating into a satellite that cooled and became the moon.
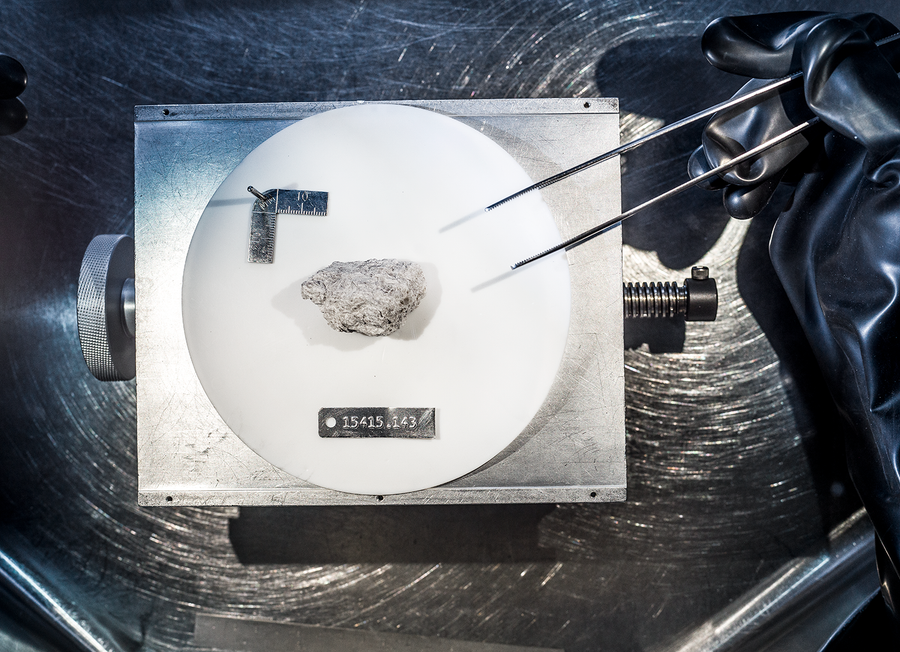
Apollo 15 sample 15415, known as the Genesis rock, which helped scientists develop the leading theory of how the moon formed. Credit: Chris Gunn
This model has been influenced by many observations from the Apollo samples and surface experiments, which include:
Iron: The moon has surprisingly little iron. Surface geophysics experiments deployed by Apollo missions showed that compared with the terrestrial planets, the moon’s core comprises a very small portion of its volume compared with the terrestrial planets—just 25 percent of its total radius. The relative lack of iron suggested by the moon’s small core is evidence that Earth had already formed an iron-rich center when the giant impact occurred, leaving little iron to form the moon.
Dryness: The lunar samples proved to be extremely dry and almost entirely depleted of volatiles—elements or molecules with low boiling points that easily evaporate, such as water, carbon dioxide, nitrogen and hydrogen. To explain this depletion, scientists suggest the massive amount of energy and heat generated from the giant impact may have driven volatiles from the fragments of the proto-moon.
Magma ocean: One of the most influential hypotheses to come from the lunar samples is the idea that there was an ocean of magma on the early moon. Apollo 11 samples showed that the lunar highlands (bright, high-standing regions as opposed to the dark lunar maria in low-lying areas) contain high concentrations of the mineral plagioclase. The texture of the rocks containing this mineral suggested that it formed from a large body of molten rock that cooled, and the light plagioclase crystals floated to the top. Because similar rocks had been found by previous robotic missions at other locations, and the lunar highlands are widespread, the layer of magma must have covered most, or all, of the moon’s surface. Two independent groups proposed the idea of this early magma ocean in 1970, just six months after the return of the first Apollo samples. Several additional lines of evidence from geochemistry and geophysics support the magma ocean model, which is still being developed today.
One piece of evidence that complicates the giant impact model is the concentration of various isotopes—atoms of an element that have a different mass from the “regular” atoms—in Apollo samples. Using a process called laser fluorination, in 2001 and 2012 researchers found that the compositions of both oxygen and titanium isotopes are almost identical between the moon and Earth. If the moon formed from a mixture of Theia and Earth materials, why does it have an Earth-like isotopic composition? This evidence has inspired new ideas, such as the “synestia” model that planetary scientists Simon J. Lock and Sarah T. Stewart describe in “Origin Story.”
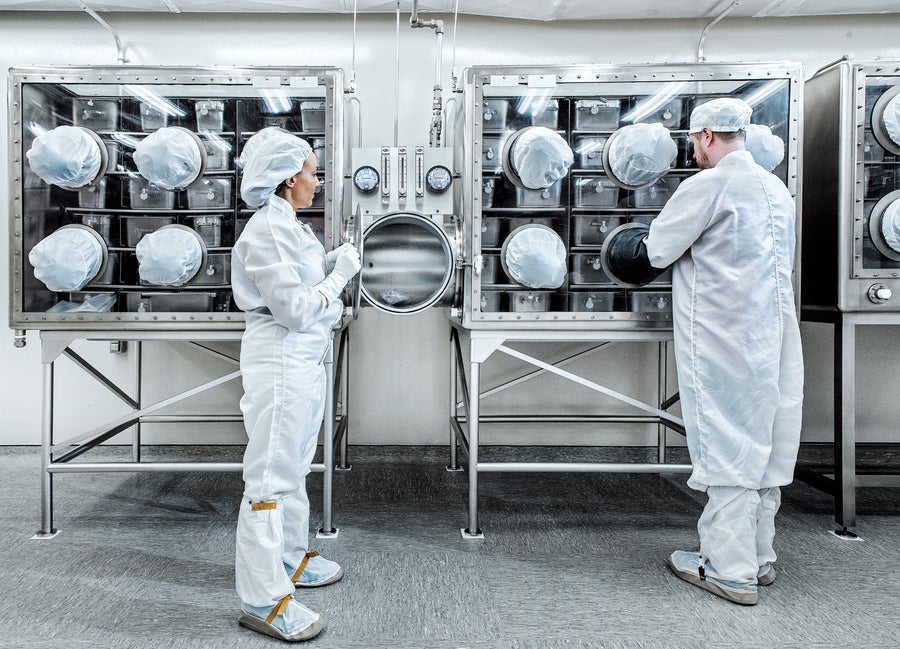
Curation processors transfer an Apollo 15 sample out of an airlock in its stainless-steel storage cabinet. Credit: Chris Gunn
The story of the solar system
Studies of lunar samples have also informed us about other planetary bodies. Perhaps the most significant result is the Nice model (so named because it was created in Nice, France) of the evolution of the solar system. According to this model, the giant planets of the outer solar system initially formed close together. After several hundred million years, their orbits became unstable, causing Saturn, Uranus and Neptune to rapidly migrate to their present-day orbits, which are much farther away from the sun. The movement of the giant planets pulled material from the outer solar system—the Kuiper belt—inward, where it collided with planets and moons and caused general chaos throughout the solar system.
This model may sound farfetched, but it elegantly explains a number of seemingly unrelated observations about our cosmic neighborhood. For instance, by dating Apollo samples and analyzing impact craters, scientists concluded that there was a cataclysmic spike in impacts on the moon about 700 million years after the planets formed, referred to as the “late heavy bombardment.” Initially there was no easy explanation for why the number of impacts would have suddenly jumped at this time. Yet the chaotic period of impacts predicted in the Nice model provides a source of impactors during the exact era in question.
In addition to telling us about the evolution of the solar system, the lunar samples have also allowed scientists to investigate the chemical evolution of planetary surfaces. “Space weathering” is a process that describes the physical and chemical erosion on bodies with no atmosphere. Studies of Apollo soils scooped from the surface showed that they contain agglutinates, welded glass and mineral fragments created by the impact of microscopic grains of dust. These agglutinates accumulate over time and can make up 60 to 70 percent of mature regolith samples. Tiny spheres of elemental iron called nanophase iron are also produced by space weathering and build up on the outer rims of certain soil grains, causing surfaces to become darker over time. We now know that solar radiation, large temperature fluctuations and the constant bombardment by tiny micrometeorites are some of the sources of space weathering.
Samples for the future
This is an exciting time in lunar science: this year caches of samples will be released that have remained unopened since they were collected almost 50 years ago on the moon. When the rocks were collected, NASA intentionally left a portion sealed to wait for technology to advance beyond the capabilities of the Apollo era. In March the Apollo Next Generation Sample Analysis (ANGSA) program selected nine research teams to receive unopened, vacuum-sealed samples from Apollo 15, 16 and 17. The opportunity to study “new” lunar samples will likely lead to more fundamental discoveries about the formation and evolution of our natural satellite.
As much as we have learned from the Apollo samples and surface experiments and as much as we will undoubtedly learn from the new caches, we desperately need more samples. For instance, we have no recognized samples from the lunar far side, the polar regions or the deep interior. Two samples I would particularly like to have are material from the South Pole–Aitken Basin, on the lunar far side, and ice from a polar crater. The South Pole–Aitken Basin is the largest recognized impact basin on the moon—and one of the largest in the solar system—and its interior could contain material from the moon’s lower crust and even its mantle. Studying the South Pole–Aitken Basin would also help us understand how extremely large basins shape the surfaces and interiors of planetary bodies. Returning a sample of lunar polar ice would tell us about the age and origin of lunar water—which, in turn, could clarify where Earth’s water originated.
These wish-list specimens could come from human exploration or robotic missions: there is no consensus among planetary scientists that either is best. Many experts argue, rightly, that robotic missions are cheaper, safer and can last longer than human missions. On the other hand, humans are more likely than robots to pick out a wider variety of unusual specimens, as evidenced by the diversity of the Apollo sample suite (rock, scooped and sieved soils, boulder chips, drill cores), sample volume and sample geology (composition, rock type, age).
The Apollo missions represent a singular accomplishment that fundamentally altered our view of the solar system. While we celebrate the 50th anniversary of humanity’s giant leap, no human has set foot on another planetary body since Harrison “Jack” Schmitt and the late Gene Cernan departed from the lunar surface, during the Apollo 17 mission, on December 14, 1972. As a scientist deeply inspired by those missions, I am actively working toward creating my generation’s Apollo moment: to see humans (people of color and of all genders) land on the surface of the moon, fueled by ingenuity, perseverance and a drive to explore the unknown.