On January 10, when Chinese researchers published the genome of a mysterious, fast-spreading, virus, it confirmed Dan Barouch’s greatest worry. The genome was similar to that of the coronavirus that caused the 2003 SARS outbreak, yet it also had striking differences. “I realized immediately that no one would be immune to it,” says Barouch, director of virology and vaccine research at Beth Israel Deaconess Medical Center in Boston.
Within days his laboratory and dozens of others around the world started designing vaccines that they hoped could protect billions of people against the SARS-CoV-2 virus, the biggest challenge to global health and prosperity since World War II. By early April almost 80 companies and institutes in 19 countries were working on vaccines, most gene-based instead of using traditional approaches, such as those that have been employed in influenza vaccines for more than 70 years. The labs predicted that a commercial vaccine could be available for emergency or compassionate use by early 2021—incredibly fast, given that vaccines to brand-new pathogens have taken a decade to be perfected and deployed. Even the Ebola vaccine, which was fast-tracked, took five years to reach widespread trials. If Barouch and his counterparts can offer a safe, effective concoction in a year, “it will be the fastest vaccine development in history,” he says.
That is a big “if,” however. Although labs have created several gene-based vaccines for other viruses, not one has been commercialized for a human illness.
On supporting science journalism
If you're enjoying this article, consider supporting our award-winning journalism by subscribing. By purchasing a subscription you are helping to ensure the future of impactful stories about the discoveries and ideas shaping our world today.
A conventional vaccine injected into the body inserts select pieces of a virus in cells near the injection site. The immune system recognizes molecules on these pieces, called antigens, as threats and reacts by making antibodies, molecules that can find the virus anywhere in the body and neutralize it. Once this dress rehearsal happens, the immune system remembers how to quash the invaders, so it can stop a future infection.
The established approach is to grow weakened viruses in chicken eggs—or more recently in mammalian or insect cells—and extract the desired pieces. The process can take four to six months to get the right antigens for familiar viruses that change every year, such as influenza. It can take multiple attempts over years for a new germ. That is far too slow to combat a virus that has already spread to pandemic proportions.
Instead labs are turning to gene-based vaccines. Scientists use information from the genome of the virus to create a blueprint of select antigens. The blueprint is made of DNA or RNA—molecules that hold genetic instructions. The researchers then inject the DNA or RNA into human cells. The cell’s machinery uses the instructions to make virus antigens that the immune system reacts to. Cells respond to the instructions as a normal part of their daily existence. This is the same trait infectious viruses exploit; they cannot reproduce on their own, so they use a cell’s machinery to make copies of themselves. They burst out of the cell and infect more cells, widening the infection.
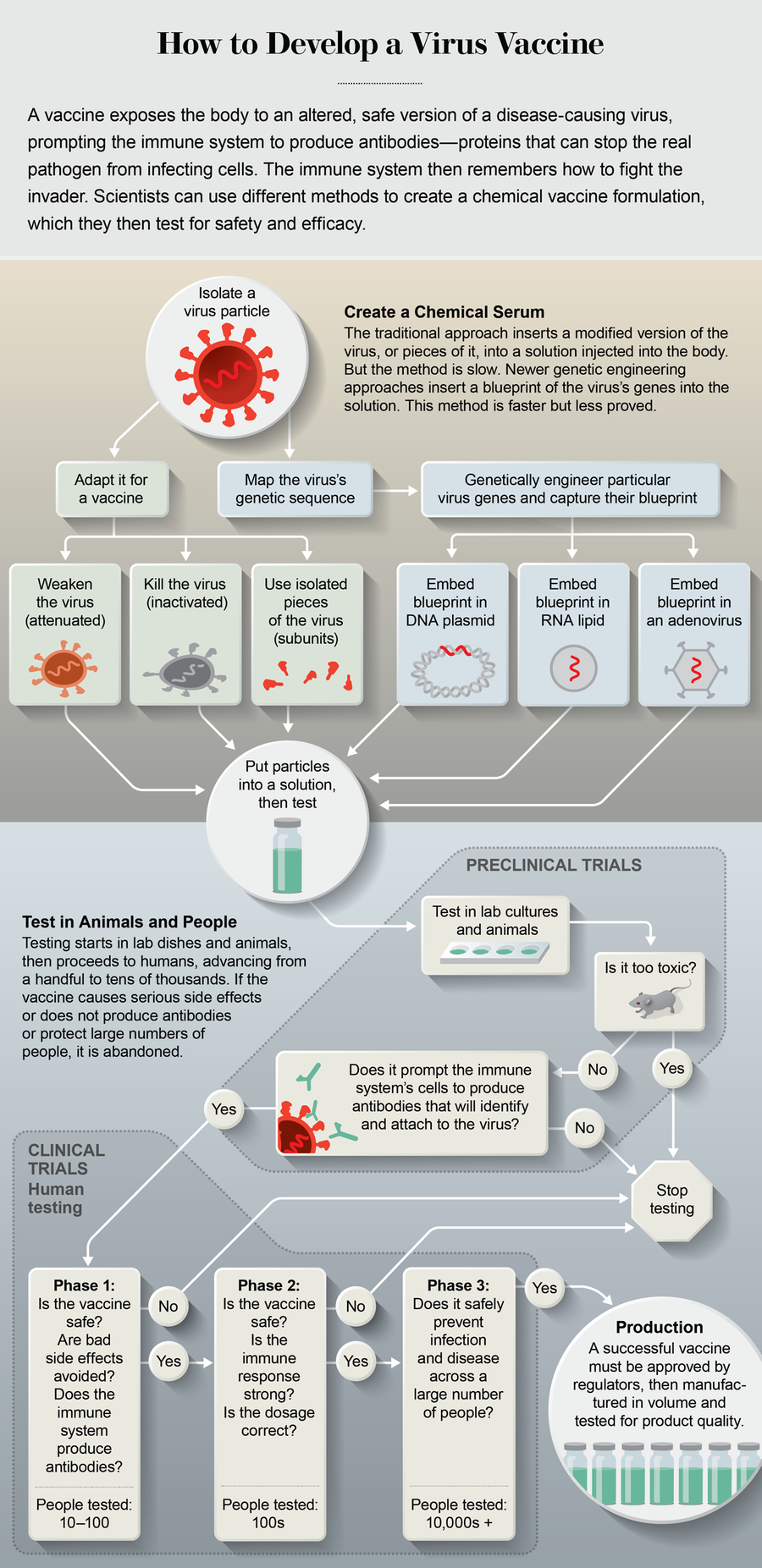
Credit: Jen Christiansen
Virtually all the labs want to find a way to train human cells to make an antigen called the spike protein. It juts out from SARS-CoV-2 like a stud on a tire, allowing the virus to bind to a human cell and sneak inside. Almost all the labs are using one of three approaches to deliver the spike blueprint. The first is a DNA plasmid, typically a small, hoop-shaped molecule. A plasmid is a handy tool because if a virus mutates, researchers can readily swap in a new blueprint. DNA-plasmid vaccines have been made for veterinary uses in fishes, dogs, swine and horses, but human applications have lagged, mostly because the vaccines have had difficulty passing through a cell’s protective outer membrane to reach the machinery inside. One recent improvement is to inject the vaccine with an instrument that administers brief electrical charges to cells near the injection site, which open pores in the cell membranes so the vaccine can enter.
Inovio Pharmaceuticals, headquartered in Plymouth Meeting, Pa., is employing the DNA-plasmid approach. Several years ago it launched clinical trials targeting spike proteins of a different coronavirus disease called Middle East respiratory syndrome (MERS). According to chief executive officer Joseph Kim, the antibody levels in vaccinated people “are as good or better than those we see in blood samples from people who [naturally] recovered from MERS.” The company adapted its platform—the plasmid and means for testing it—to make a vaccine for SARS-CoV-2.
DNA-plasmid vaccines work by transferring the genetic blueprint to RNA in the cell machinery, which makes the spike antigens. But scientists can skip the plasmid step by embedding a blueprint in a strand of RNA—a second approach known as RNA vaccines. The RNA is carried in lipids that are injected into the body; lipids are fatty molecules that can pass easily into cells. Research shows that RNA vaccines may be better than DNA plasmids at mobilizing the immune system to create antibodies. They also seem to induce more potent immunity—a stronger memory in the immune system—and therefore require lower doses. Some RNA vaccines are in early-stage clinical trials for other viral illnesses, including rabies, HIV and Zika. Moderna in Cambridge, Mass., is using this approach for SARS-CoV-2.
RNA vaccines are less stable than DNA-plasmid vaccines; common enzymes in the body can quickly degrade them. Heat can ruin them, too. RNA vaccines must generally be kept frozen or refrigerated, which creates logistical hurdles, particularly in poorer countries. DNA-plasmid vaccines are stable at higher temperatures.
Barouch and his collaborators at Johnson & Johnson are using a third approach: inserting the DNA blueprint into a common cold virus. When injected, this adenoviral vector, as it is called, infects human cells and delivers the blueprint it is carrying. Adenoviruses are good at getting into cells, yet past work shows that the human immune system readily recognizes some adenoviruses and attacks them before they can sneak in. Barouch is using an adenovirus that testing shows is unlikely to be recognized. Some experts also worry an adenovirus itself could replicate inside the body and cause disease. To address that possibility, Barouch’s team is using an engineered virus that is nonreplicating—it cannot make copies of itself inside a human cell, because it needs a substance for replication the human body does not provide. In late April the University of Oxford began a limited human trial with another nonreplicating adenovirus.
Once a vaccine’s basic functionality is confirmed in lab cultures, it is assessed in animals to see if it is safe and whether it elicits an immune response. Next it is tested in people—first small groups to check on safety and side effects, then increasingly larger numbers to see how effective it is. Inovio’s DNA plasmid went into small-scale human trialson April 6—only three months after the SARS-CoV-2 genome was published. Moderna began small human trials of its RNA vaccine even sooner, on March 16, and in April the U.S. government pledged up to $483 million to speed mass production if the trials go well. Barouch’s lab devised a prototype adenovirus vaccine in just four weeks. Johnson & Johnson, in collaboration with Barouch’s lab, is now testing it in mice, ferrets and rhesus macaques. On March 30 the U.S. and Johnson & Johnson committed more than $1 billion to fund large human clinical trials, which are slated to begin in September if the limited testing proves out.
Although the time from outbreak to small tests has been far quicker than it would have been using the egg approach, there is no guarantee that the extended trials of genetically engineered vaccines will not take years. Fortunately, SARS-CoV-2 does not appear to mutate as quickly as influenza, suggesting that an effective vaccine, once developed, might offer protection for a long time.
In addition to efficacy, the experts are watching the trials for “disease enhancement”—the possibility that a vaccine might inadvertently worsen symptoms of COVID-19, the disease that SARS-CoV-2 causes. Ferrets given an experimental SARS vaccine in 2004 developed damaging inflammation. Kim says humans who were treated with the experimental SARS vaccines did not experience disease enhancement. But those formulations never made it to large-scale human trials because the outbreak—which sickened about 8,000 people in nearly 30 countries—burned out in just over a year.
Companies are accelerating the development time for a SARS-CoV-2 vaccine in part by testing vaccines in multiple animal species at once and in parallel with small numbers of people. Usually the process is one animal at a time, and people later, to make sure that side effects are small, that immune response is large and that disease is actually defeated. Lack of time warrants greater risk.
Protecting the globe against COVID-19 will require enormous manufacturing capacity. The DNA-plasmid and RNA vaccines have never been scaled up to millions of doses, and small firms such as Inovio and Moderna would not have such capacity in-house. According to Barouch, the adenovirus vaccine is more time-consuming at the outset, but once proved it “can be scaled up quickly.” Johnson & Johnson used an adenovirus approach to generate millions of doses of a vaccine against Ebola, which are now in widespread human trials. A few groups are investigating other DNA techniques that could take longer.
No prototype vaccine is a clear favorite yet, according to Brenda G. Hogue, a virologist and coronavirus expert at Arizona State University. But she says the speed of the genetics work and the full weight companies are throwing behind it are encouraging: “I feel very positive.”
Read more about the coronavirus outbreak from Scientific American here. And read coverage from our international network of magazines here.