In October 2022 a bird with the code name B6 set a new world record that few people outside the field of ornithology noticed. Over the course of 11 days, B6, a young Bar-tailed Godwit, flew from its hatching ground in Alaska to its wintering ground in Tasmania, covering 8,425 miles without taking a single break. For comparison, there is only one commercial aircraft that can fly that far nonstop, a Boeing 777 with a 213-foot wingspan and one of the most powerful jet engines in the world. During its journey, B6—an animal that could perch comfortably on your shoulder—did not land, did not eat, did not drink and did not stop flapping, sustaining an average ground speed of 30 miles per hour 24 hours a day as it winged its way to the other end of the world.
Many factors contributed to this astonishing feat of athleticism—muscle power, a high metabolic rate and a physiological tolerance for elevated cortisol levels, among other things. B6’s odyssey is also a triumph of the remarkable mechanical properties of some of the most easily recognized yet enigmatic structures in the biological world: feathers. Feathers kept B6 warm overnight while it flew above the Pacific Ocean. Feathers repelled rain along the way. Feathers formed the flight surfaces of the wings that kept B6 aloft and drove the bird forward for nearly 250 hours without failing.
One might expect that, considering all the time humans have spent admiring, using and studying feathers, we would know all their tricks by now. Yet insights into these marvelous structures continue to emerge. Over the past decade other researchers and I have been taking a fresh look at feathers. Collectively we have made surprising new discoveries about almost every aspect of their biology, from their evolutionary origins to their growth, development and aerodynamics.
On supporting science journalism
If you're enjoying this article, consider supporting our award-winning journalism by subscribing. By purchasing a subscription you are helping to ensure the future of impactful stories about the discoveries and ideas shaping our world today.
Among the creatures we share the planet with today, only birds have feathers. It makes sense, then, that for centuries scientists considered feathers a unique feature of birds. But starting in the 1990s, a series of bombshell fossil finds established that feathers were widespread among several lineages of the bipedal, carnivorous dinosaurs known as theropods and that birds had inherited these structures from their theropod ancestors. The discovery of feathered nonbird dinosaurs sent researchers scrambling to understand the origin and evolution of feathers, especially their role in the dawn of flight. We now know many dinosaurs had feathers, and protofeathers probably go all the way back to the common ancestor of dinosaurs and their flying reptile cousins, the pterosaurs. Bristles, fuzzy coverings, and other relatively simple featherlike structures probably decorated a wide array of dinosaurs—many more than we have been lucky enough to find preserved as fossils.
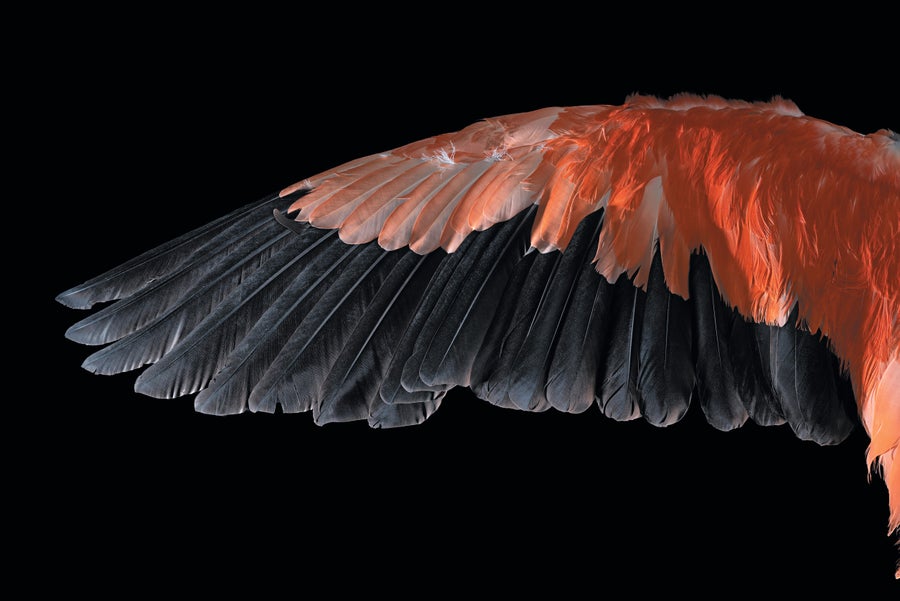
Feathers, such as those of the Lesser Flamingo shown here, are products of hundreds of millions of years of evolution. Robert Clark
The feathers on nonbird dinosaurs were not limited to bristles and fuzz, however. The flat, broad, flight-enabling feathers we see across most of the wings and much of the body surface of living birds are called pennaceous feathers. (Fun fact: these are the feathers people used to make into quills for writing, hence the word “pen.”) It turns out that these feathers, too, appeared before birds. In fact, there is an entire group of dinosaurs comprising birds as well as species such as Velociraptor that takes its name from these very feathers: the pennaraptoran clade. Fossils of early pennaraptorans show that they had feathery coverings that would have looked essentially modern at a quick glance.
The flight capacity of these early pennaraptorans has been hotly contested. Some species were clearly not fliers, given the small size of their “wings” relative to their large bodies. For those animals, pennaceous feathers were probably display pieces. But other pennaraptorans, such as the small, four-winged, forest-dwelling Microraptor, are trickier to interpret. Many of the arguments about whether this creature could fly have centered on something called vane asymmetry. The two flat “blades” of a feather on either side of the main shaft are called vanes. In living birds that fly, the feathers that arise from the hand, known as the primaries, have asymmetrical vanes: the leading vane is narrower than the trailing one. It stood to reason that vane asymmetry was important for flight. And because fossils of Microraptor and its kin show asymmetrical feathers, some researchers argued, these animals must have been able to fly.
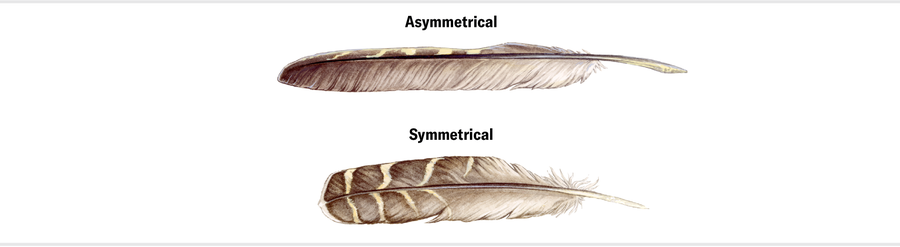
Maria Amorette Klos
Recent work by flight biomechanics experts, including me, has overturned this received wisdom about feather vane asymmetry. Our research shows that feather shape is largely optimized to allow the feather to twist and bend in sophisticated ways that greatly enhance flight performance. Merely being anatomically asymmetrical doesn’t mean much. What matters is that the feather is aerodynamically asymmetrical, and for this to be the case, the vane asymmetry must be at least three to one—that is, the trailing blade needs to be three times wider than the leading one. Below this ratio, the feather twists in a destabilizing rather than stabilizing way during flight.

Maria Amorette Klos
Early pennaraptorans such as Microraptor didn’t have aerodynamically asymmetrical feathers. But that doesn’t mean they couldn’t fly. The tendency to twist (whether in a stabilizing or a destabilizing fashion) is only relevant if the feathers are separated enough to do so. Keeping feathers in a wing tip tight and overlapping makes them stable, even if they’re not asymmetrical. Asymmetry matters only if the flier spreads its primaries apart in flight like many modern raptors do—a feature called slotting. So Microraptor and its kin could probably use flapping flight, but their wing shape was necessarily different from that of today’s forest-dwelling birds of prey. Specifically, Microraptor had relatively long, narrow wings with tight, unslotted wing tips—anatomically distinct from the wings of Cooper’s Hawks and other modern-day forest hawks but aerodynamically similar.
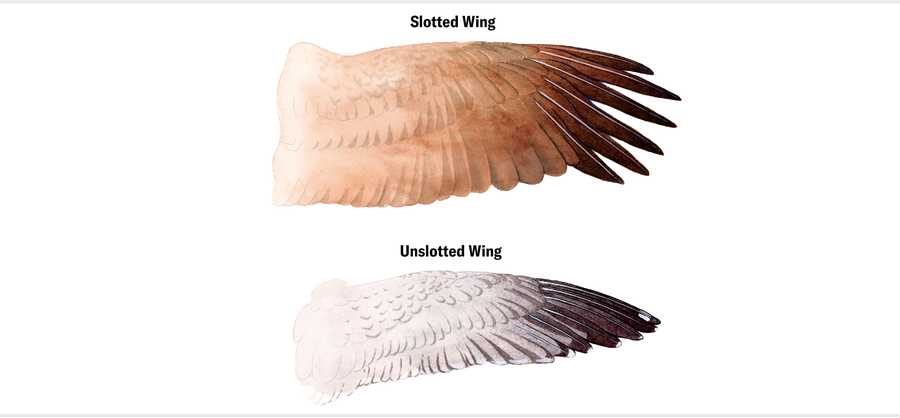
Maria Amorette Klos
After considering these findings on vane asymmetry, as well as new data on flight muscles in near-bird dinosaurs, a group of researchers (of which I was the senior biophysicist) led by Michael Pittman of the Chinese University of Hong Kong recently concluded that powered flight—that is, flapping flight rather than gliding flight—probably evolved multiple times in dinosaurs, with just one of those lineages surviving to the present in the form of birds. Yet only in birds did flight feathers attain the degree of shape-shifting we see today. That ability of feathers to twist in just the right way is what enabled slotting, which makes the wing much more efficient at low flight speeds. In essence, a slotted wing behaves as if it is longer and narrower than it is anatomically. Slotting also makes the wing tip very resistant to stall, whereby the airflow separates from the wing, causing a precipitous loss of the lift that keeps the bird in the air. It’s a vital adaptation that underpins an array of aerial acrobatics.
Birds typically need long, narrow wings to soar efficiently—seabirds such as albatrosses and petrels are perfect examples. The advent of wing-tip slots made it possible to soar with broader wings, paving the way for evolution of a diversity of broad-winged soarers, including vultures and hawks. The aerodynamic advantages of slotting also permit the explosive flight performance of sprinters such as grouses, which spend most of their time on the ground but burst into flight for a short distance when startled. And wing-tip slots provide much greater maneuverability for a wide array of birds that live in forests and other cluttered environments, from songbirds to toucans. In fact, the maneuverability made possible by slotted wings might have helped birds compete with pterosaurs and ultimately survive the end-Cretaceous extinction.
The pennaceous feathers we associate with flight aren’t the only type of feather birds possess. Feathers in different regions of the body vary in size, shape and function. You can think of feather form as a spectrum, with the large, relatively stiff flight feathers of the wing and tail at one end and the short, fluffy down feathers that sit close to the body at the other. All of them have a central shaft and softer “barbs” that branch out from the shaft. In flight feathers, the barbs interlock like Velcro teeth to form the smooth, windproof surface of the vanes. In down feathers, the barbs are loosely structured and fluffy to trap heat. Many of the other kinds of feathers combine aspects of these two types. The contour feathers that streamline a bird’s body, for example, have vaned tips like flight feathers and noninterlocking barbs like down ones. The bristle feathers that typically occur on the face and may serve protective and sensory purposes meld the flight feathers’ stiff shafts (called rachises) with the down feathers’ fluffy base.
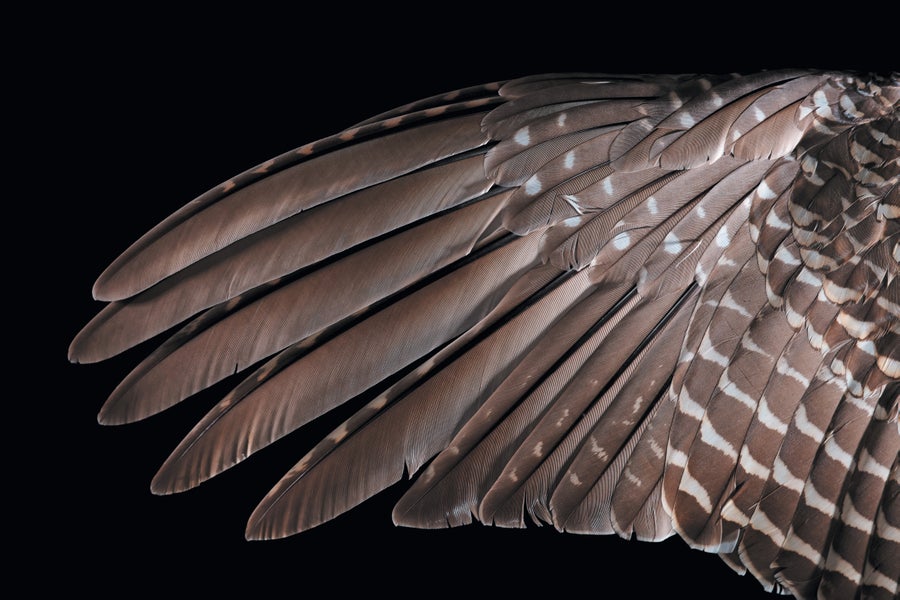
The wing of the Greater Prairie-Chicken, a type of grouse, has a slotted tip that helps the bird burst into flight when startled.
Robert Clark
In recent years researchers have begun to piece together the intricate process by which feathers develop. Like scales, spines and hairs, feathers are skin appendages. Scientists have known for a while that they arise from structures in the skin. But how can an animal produce feathers with different anatomies across its body?
My colleagues and I, led by Cheng-Ming Chuong of the University of Southern California, related the developmental biology of various kinds of pennaceous feathers to their mechanical properties. These feathers begin as a tube that essentially unzips along its length, forming the two vanes. Several genes and molecules interact with one another and with the environment to determine the amount of interlocking in the barbs that make up the vanes, the size and shape of the rachises, and whether the shaft is filled with a “foam” that makes it stiff relative to its weight. We found that different feather types have varying specializations in their overall stiffness, their tendency to twist, and the distribution of the foam in the shaft. These variations depend to some extent on the work of different genes, but most of the differentiation is the result of changes in how the genes are regulated—that is, when they are turned on or off or how active they are during feather development.
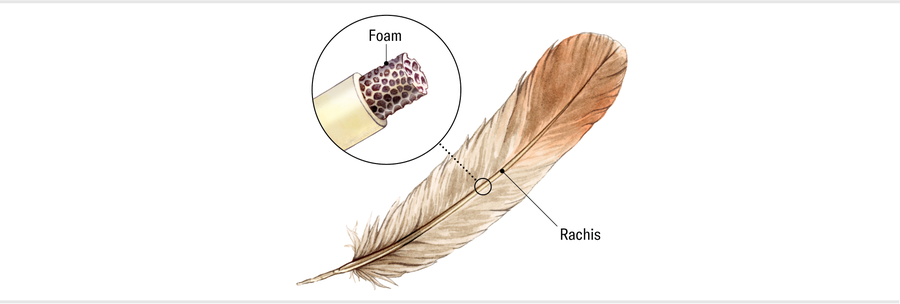
Maria Amorette Klos
Scientists have also shown a recent surge of interest in another category of feathers: display feathers, the showy feathers that help to attract mates. Display feathers may dazzle an observer with their colors (think of a hummingbird’s glittering throat), or they may attain eye-catching proportions, like the feathers that make up a peacock’s crest and train. The conventional wisdom about display feathers holds that they are strictly products of sexual selection, in which mate choice drives the evolution of a trait. These days, however, researchers around the globe, me included, are coming to see display feathers not as exclusively sexually selected traits with no mechanical properties of interest but instead as complex compromises between the pressures of social biology and mechanobiology.
To wit: long display feathers don’t grow just anywhere on the body. They most often occur on the lower back and tail, where they interfere comparatively little with flight performance. Take, for example, the Resplendent Quetzal, a small, colorful bird native to the cloud forests of Mexico and Central America with tail feathers that can grow up to three feet long on males during the breeding season. The tail streamers might not be shaped solely by sexual selection. Evidence indicates that the streamers of some birds produce at least a little aerodynamic force, enough to support much of their added weight. The quetzal’s streamers, for their part, lost their tight interlocking structure, making the vanes a pennaceous-downy hybrid that lets much of the airflow pass through without producing much lift. This arrangement is most likely an adaptation to prevent these feathers from being highly destabilizing. These flashy feathers still increase the cost of flying because they add drag, but that cost may well be less than has been assumed.
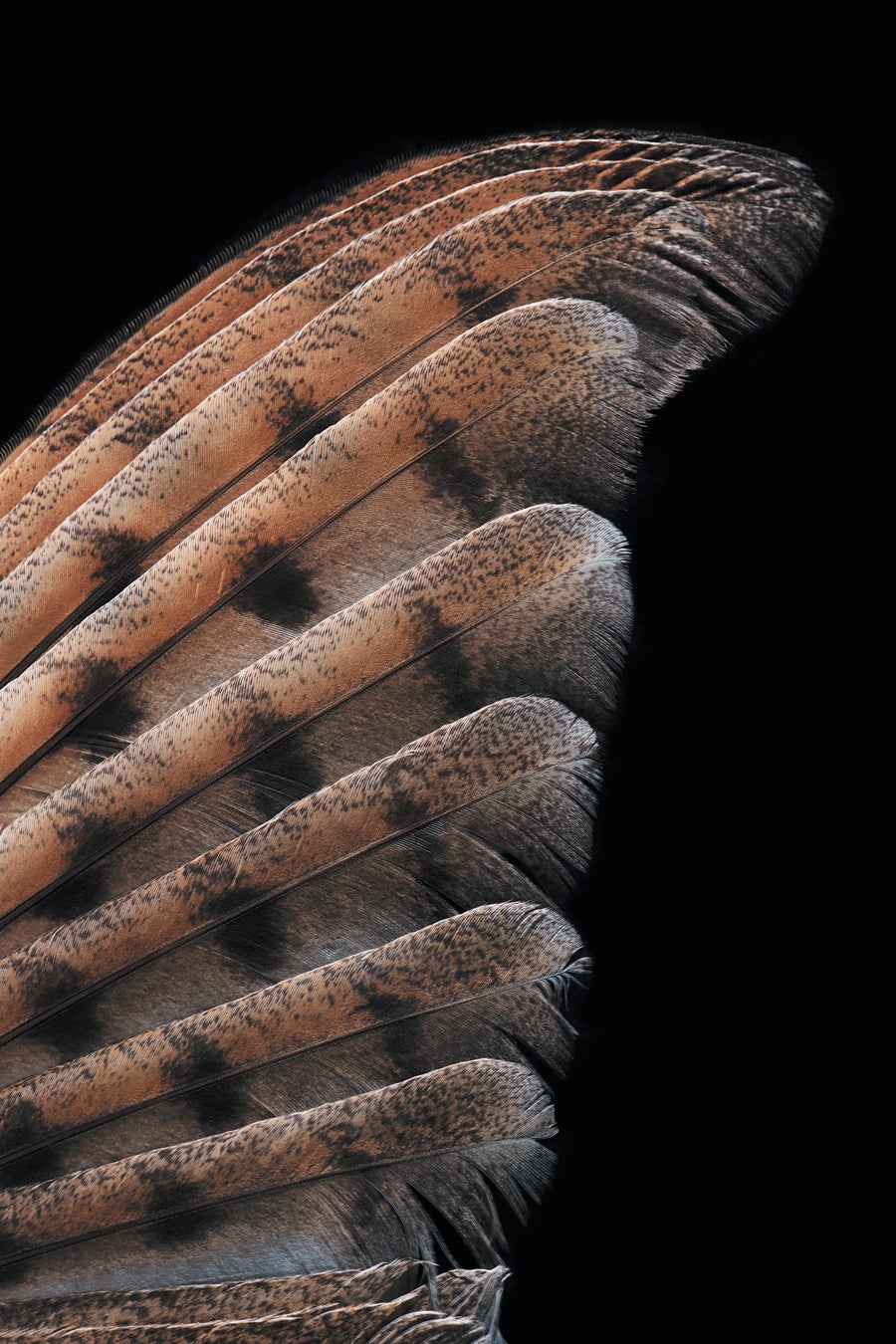
The Barn Owl‘s primary feathers have features that allow this bird of prey to fly silently.
Robert Clark
The microstructure of display feathers, especially tail streamers, may also be more finely tuned than previously thought. Feather structure provides a balance of stiffness, weight and shape. The feathers must hold their shape well enough, even at extreme lengths, to be effective signals. But they cannot be so stiff as to destabilize the bird during gusty winds or tight maneuvers. There’s a particular range of flexibility that shows off the feather to best effect while minimizing detrimental impacts on flight performance.
One of the aspects of feathers that has long fascinated me is their adaptability. Under varying conditions and evolutionary pressures, they can become specialized for everything from speed and maneuverability to insulation or display. Some of the most fascinating adaptations can be found in owls.
Facial disks are an especially conspicuous feature of owls. These broad, semicircular fans of feathers around the eyes and ears give owls their distinctive appearance. The skull of an owl is actually quite long and narrow, but the feathers enveloping it completely change the contours of the animal. These facial disks are not just for looks. They do a remarkably good job of funneling sound to the owl’s ears. The disks, along with vertically offset ears and exceptionally sensitive middle and inner ear structures, make owls so good at determining the origin of a sound that they can zero in on prey without seeing it at all (they still use vision to make the final capture, though).
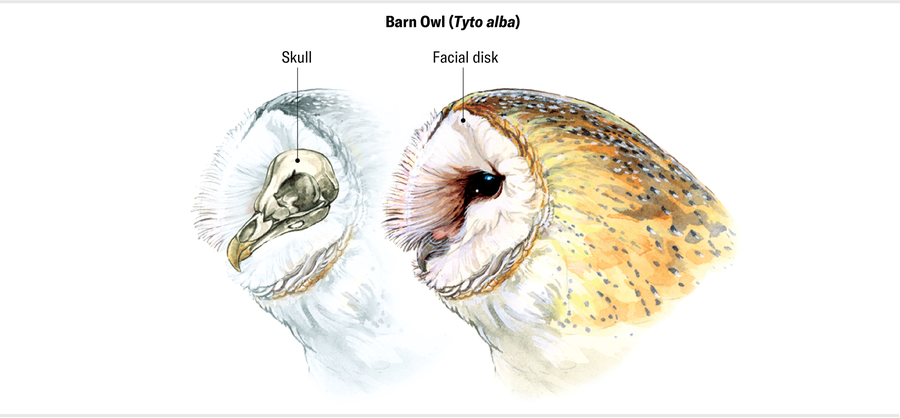
Maria Amorette Klos
I have worked with quite a few owls over the years, particularly individuals being rehabilitated after injury. One such owl couldn’t be released because a car strike had left him completely blind. Yet if someone tossed food onto one of his perches, the gentle thud of it landing was enough for him to pounce on it perfectly. (Readers may also find solace in knowing that he still flew, having memorized his enclosure, and was regularly taken around for walks and neck scratches.)
Still, that exceptional sense of hearing wouldn’t get owls very far without some additional feather adaptations. Other nocturnal creatures can also hear very well, and an owl whose feathers were rustling in flight would be hard-pressed to get close to its vigilant prey. Furthermore, owls might not hear quietly creeping prey if their own feather sounds covered the faint noises of their targets. Owls solved both problems by evolving feather traits that make them inaudible during flight.
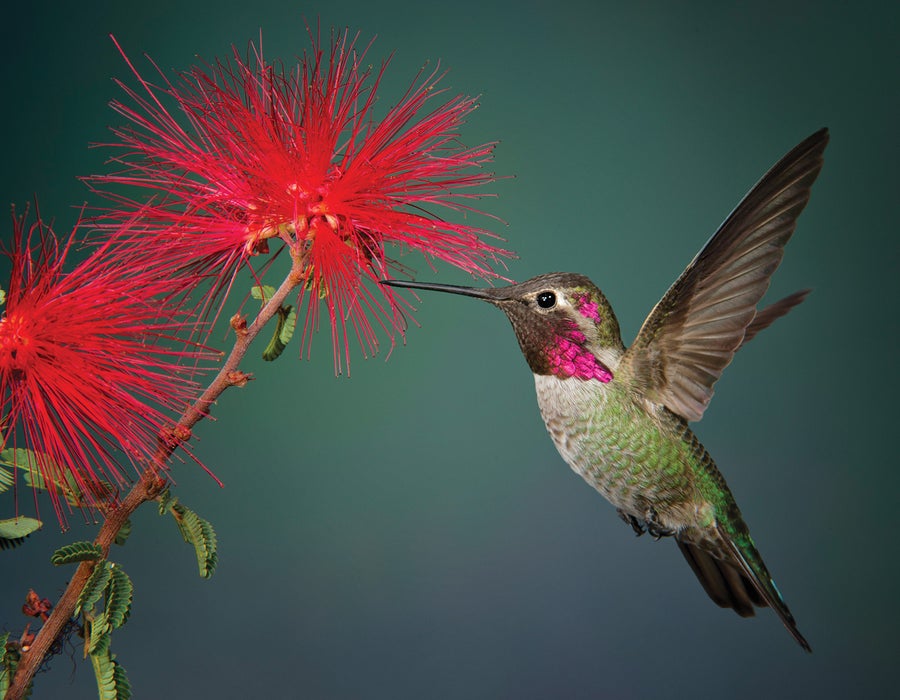
The extremely stiff feathers of hummingbirds such as Anna’s Hummingbird help to support their distinctive, hovering flight.
Kathleen Reeder Wildlife Photography/Getty Images
It is hard to appreciate just how quiet owls are. Even ultrasensitive microphones, if properly calibrated, aimed exactly right and set to maximum sensitivity in a silent space, can just barely pick up sounds from a flying owl ... sometimes. For all practical purposes, owls are silent. They are so eerily noiseless that even if they fly over your head close enough for you to feel their wake, you will still hear absolutely nothing. In a dark space, they are essentially undetectable. All the owl wing sounds you hear in the Harry Potter movies and other films? Those are added in.
Owls achieve this stealth with a few different feather adaptations. To start, their feathers have a “velvety” surface that silences them when they move against one another. More important, the feathers on the leading edge of an owl’s wing have a set of comblike structures, whereas those on the trailing edge have fluffy fringes. The leading-edge comb stirs the air in a specific way called micro vorticity. These tiny, swirling streams of air cause the main flow to stick to the wing. In aerodynamic speak, we say the combs “inject vorticity into the boundary layer.” When this modified flow then passes through the trailing-edge fringes, the net result is a wake that contains no coherent waves of linear pressure and therefore no sound. Put another way, there are no vibrations from the interactions between feathers and the air capable of producing sound.
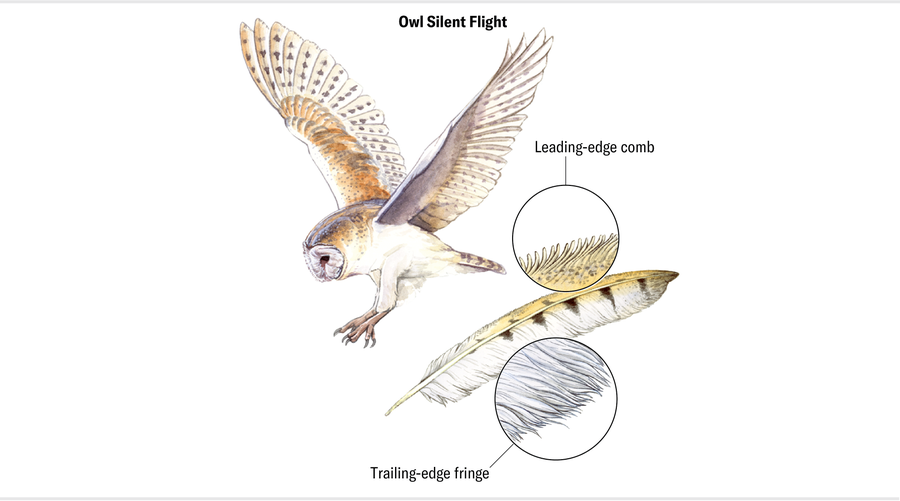
Maria Amorette Klos
These specializations have deep roots. Modern-day owls belong to one of two groups: the tytonids (represented by Barn Owls and Bay Owls) and the strigids (all other living owls). Their last common ancestor existed at least 50 million years ago. Because owls in both groups exhibit silent flight, this trait probably dates to their common ancestor. In other words, owls have been surreptitiously coursing the night skies for more than 50 million years.
Not surprisingly, some of the most extreme feather adaptations are found in birds with the most extreme ecological specializations. One way feathers can adapt to a particular way of life is by increasing or decreasing in stiffness. Coincidentally, the stiffest feathers are found in two groups of birds that are otherwise as different as can be: hummingbirds and penguins.
Hummingbirds have ultrastiff feathers as an adaptation to the exceptionally high flapping frequencies and unusual flapping stroke they use to hover in front of flowers while sipping nectar. Unlike most birds, hummingbirds can get a substantial amount of weight support and thrust from their upstroke, not just their downstroke. They do this by rotating their shoulder to flip the wing over completely. The wing needs to be very stiff for this method to work. Reinforcements in the bones of the hummingbird wing provide some of this rigidity; feathers with extremely firm rachises provide the rest.
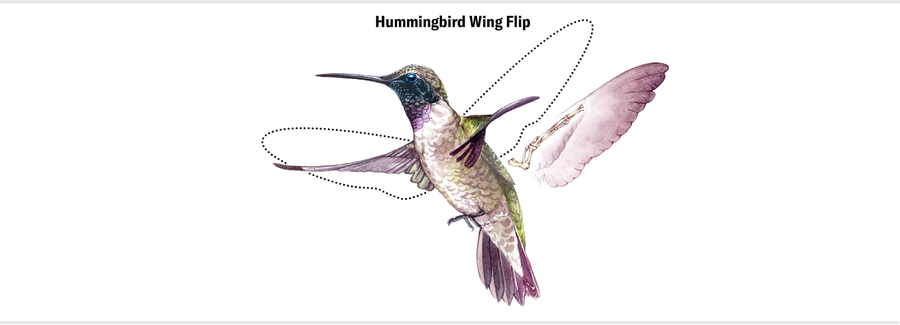
Maria Amorette Klos
The flightless penguins, in contrast, have adapted to life in the water and on land. They possess some of the most specialized plumage of all, having converted their entire body covering into a densely packed mosaic of tiny feathers. These feathers are individually quite stiff, and together they form a textured surface over the wings and body that regulates the boundary layer of water against them while the penguin is swimming. In essence, they use a rough coat of feathers to catch and hold a smooth jacket of water. The net effect is a reduction in drag and therefore a lower energetic cost of swimming. The dense feathers also trap just enough air to provide some insulation without making the penguin buoyant, supplementing the fat layer that helps to keep the bird warm.
In the absence of any constraints posed by flight, penguins jettisoned the more typical feather accoutrements of their ancestors in favor of a novel suit of drag-reducing, minimum-buoyancy feathers. These feathers are a key part of the package of adaptations that have made penguins the undisputed diving champions of the avian world, capable of reaching depths of more than 1,600 feet in search of krill, fish, and other aquatic prey.
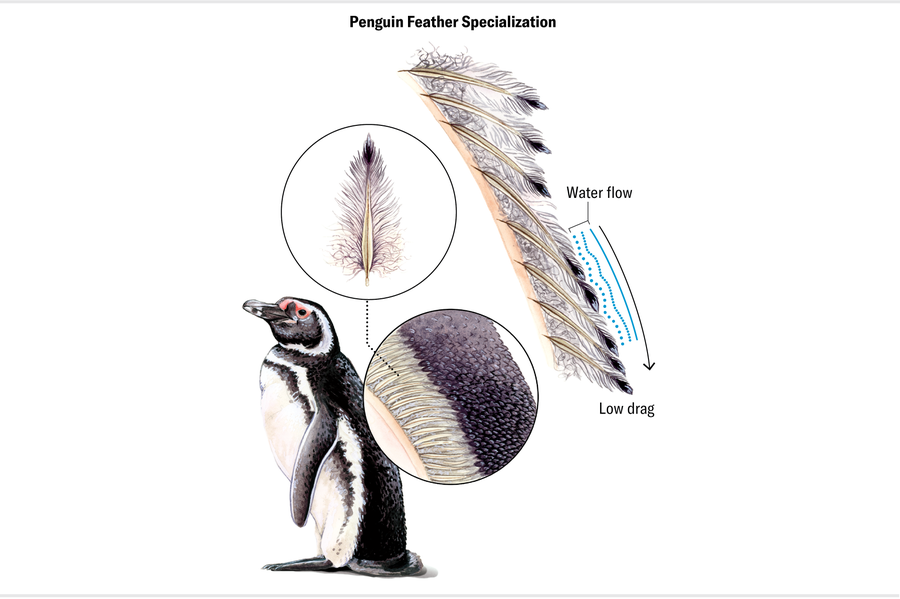
Maria Amorette Klos
Feathers are a fantastic model system for understanding how complex structures evolve and how anatomy and behavior influence each other over time. It’s no wonder that the applied science sector has taken note of feathers’ many brilliant features. Already they have led to successful technological innovations. The Velcro-like mechanism that connects the barbs of pennaceous feathers is the basis for an advanced temporary fastening system. The silencing fringes of owl feathers have inspired ventilation-quieting systems. The surface texture and boundary-layer-control principles of penguin feathers have made their way into robotics, mostly in prototypes.
No doubt feathers will give rise to more clever inventions in the future. We have only to let our creativity take flight.
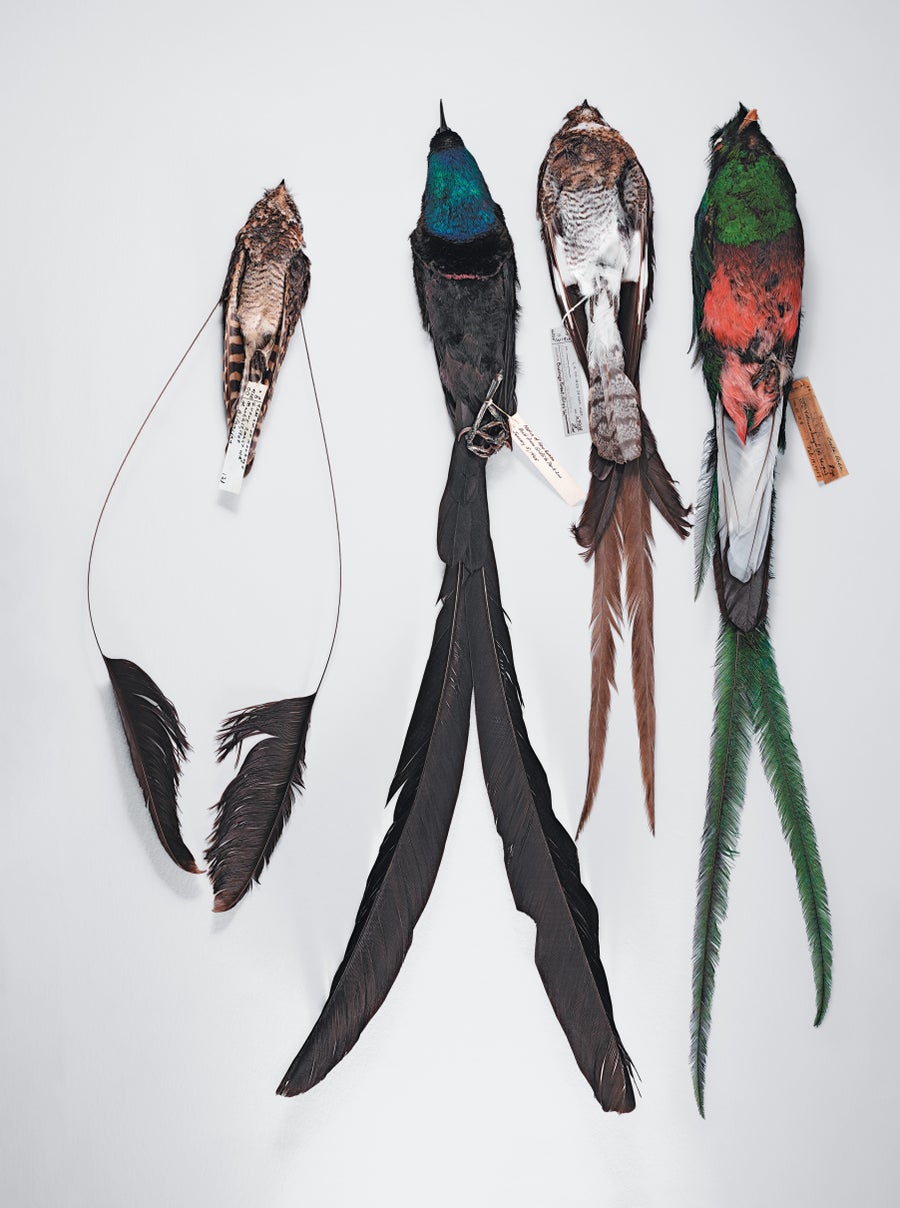
Long display feathers may be present on the wings, as happens in the Standard-winged Nightjar (left) and Pennant-winged Nightjar (right center). But they usually grow on the lower back and tail, which minimizes any negative impact on flight, as in the Stephanie’s Astrapia (left center) and Resplendent Quetzal (right).
Robert Clark