Deep in the heart of the Milky Way, strange things happen. This is a place where stars slingshot around apparently empty space at an appreciable fraction of the speed of light. Scientists have long thought that only a supermassive black hole could explain the stars’ movements, but until very recently, they hesitated to say that outright. For example, when astronomers Reinhard Genzel and Andrea Ghez shared a portion of the 2020 Nobel Prize in Physics, their citation specified that they were awarded for “the discovery of a supermassive compact object at the centre of our galaxy,” not the revelation of a “black hole.” The object is known as Sagittarius A* (“Sagittarius A star”).
In 2022, however, the astronomers behind the Event Horizon Telescope (EHT) settled the matter by unveiling the first image of a supermassive black hole at the center of the Milky Way. It wasn’t the first picture of a black hole this collaboration had captured—that was the iconic image of M87*, which they revealed in April 2019. But it was the one they wanted most. Sagittarius A* is our own private supermassive black hole, the still point around which our galaxy revolves.
Black holes trap everything that falls in, including light, so they are, in a very real sense, unseeable. But they warp spacetime around them so severely that, when they are illuminated by glowing streams of infalling matter shredded in their gravitational grip, they cast a “shadow.” The shadow is about two and a half times larger than a black hole’s event horizon, the boundary in spacetime through which nothing that passes can ever return.
On supporting science journalism
If you're enjoying this article, consider supporting our award-winning journalism by subscribing. By purchasing a subscription you are helping to ensure the future of impactful stories about the discoveries and ideas shaping our world today.
The EHT captured images of this shadow by using a technique called very long baseline interferometry (VLBI), which combines radio observatories on multiple continents to form a virtual Earth-size telescope, an instrument with the highest resolution in all of astronomy. In April 2017 the EHT collaboration spent several nights pointing that virtual instrument at Sagittarius A* and other supermassive black holes. The scientists then spent years analyzing the raw data and converting them into an image.
Part of the reason it took so long was the global devastation of the COVID pandemic. But the bigger challenge was that Sagittarius A* is constantly changing. The observatory’s previous target, M87*, the black hole at the heart of the galaxy Messier 87 (M87), is so huge that the matter swirling around it takes many hours to complete a full orbit. Practically speaking, that means you can stare at it for a long time, and it will scarcely change. The mass of Sagittarius A* is less than one one-thousandth of M87’s, so its appearance changes about 1,000 times faster, as matter moves in tighter, quicker orbits around the black hole. Katie Bouman, a California Institute of Technology computer scientist and astronomer who co-leads the EHT’s imaging working group, said that matter orbits Sagittarius A* so quickly that it changes “minute to minute.” Imagine taking a time-lapse photograph of a speeding bullet—it’s not easy.
If Sagittarius A*’s mercurial nature made it hard to see, it also makes it an ideal laboratory for understanding black holes and Einstein’s general theory of relativity, his hallowed theory of gravity. Through decades of study with all manner of telescopes, astronomers already knew Sagittarius A*’s basic measurements (its mass, diameter and distance from Earth) to great accuracy. Now, at last, they’ve gained the ability to watch it evolve—to watch as it feeds on flaring, flashing streams of matter—in real time.
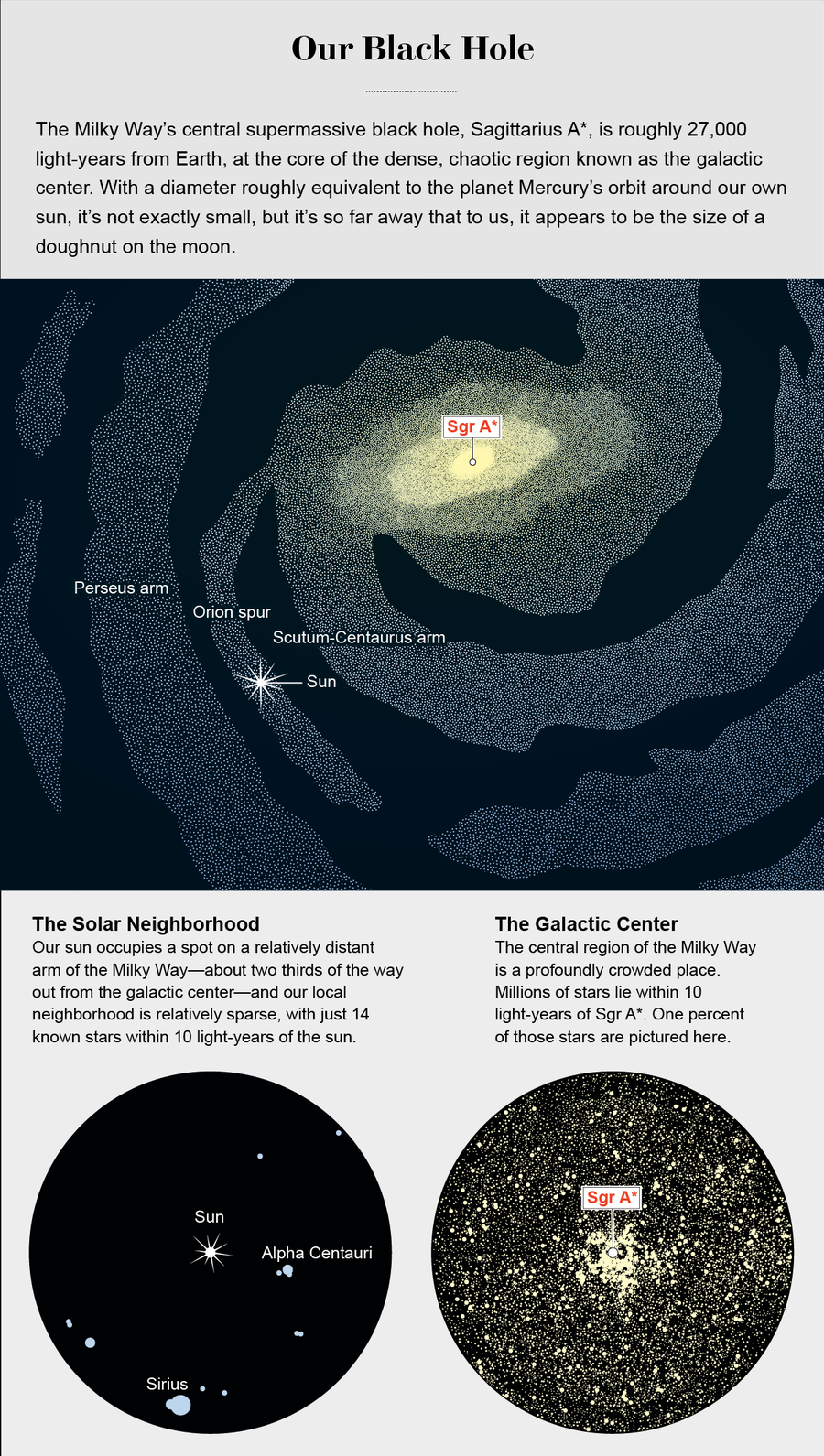
Katie Peek; Source: Bob Benjamin, University of Wisconsin–Whitewater (Milky Way structure)
Scientists started to suspect that a black hole lurked in the heart of the Milky Way in the early 1960s, not long after the discovery of active galactic nuclei—extremely bright regions at the cores of some faraway galaxies illuminated by voraciously feeding supermassive black holes. From our perspective here on Earth, active galactic nuclei are a thing of the past—we see them only in the distant, ancient universe. Where did they all go? In 1969 English astrophysicist Donald Lynden-Bell argued that they didn’t go anywhere. Instead, he said, they just went to sleep. Dormant supermassive black holes, he predicted, are slumbering all around us in the hearts of nearly all spiral galaxies, including our own.
In 1974 American astronomers Bruce Balick and Robert Brown pointed radio telescopes in Green Bank, W.Va., at the center of the Milky Way and discovered a dim speck they suspected was our galaxy’s central black hole. They found it in a slice of sky, known as Sagittarius A, within the constellation Sagittarius. Radiation from the new source was lighting up—or “exciting”—surrounding clouds of hydrogen. Brown borrowed from the nomenclature of atomic physics, in which excited atoms are marked with an asterisk, and named the newfound speck Sagittarius A*.
For the next two decades radio astronomers kept gradually improving their view of Sagittarius A*, but they were limited by a lack of suitable telescopes, relatively clunky technology (think reel-to-reel magnetic tape) and the inherent difficulty of looking into the crowded galactic center.
Sagittarius A* is concealed by a multilayered veil. The first layer is the galactic plane—27,000 light-years’ worth of stars, gas and dust that blocks visible light. Radio waves sail through the galactic plane unimpeded, but they’re obscured by the veil’s second layer—the scattering screen, a turbulent patch of space where density variations in the interstellar medium knock radio waves slightly off course. The final layer concealing Sagittarius A* is the infalling matter surrounding the black hole itself. Peering through that barrier is a bit like peeling off an onion’s skins. The outer layers of matter emit longer-wavelength light—the same wavelengths VLBI traditionally works with. Making VLBI work with shorter-wavelength light would enable closer-in views approaching the black hole’s event horizon, but it was a major technological challenge.
For a while, astronomers using other techniques besides VLBI had more success, steadily gathering indirect evidence that Sagittarius A*’s “speck” was actually a seething supermassive black hole. In the 1980s physicist Charles Townes and his colleagues showed that gas clouds in the galactic center were moving in ways that made sense only if they were under the influence of some great, unseen gravitating mass. And in the 1990s Ghez and Genzel independently began tracking the orbits of giant blue stars in the galactic center, mapping their motion around a heavy but hidden pivot point.
Meanwhile the situation for radio astronomers improved. In the late 1990s and early 2000s a new generation of short-wavelength radio telescopes started to come online—telescopes that, if augmented with lots of bespoke equipment, could participate in VLBI observations at the microwave frequencies thought to shine from the edge of Sagittarius A*’s shadow. At the same time, the computing revolution that led to solid-state hard drives and smartphones in every pocket vastly increased the amount of data that each observatory in a network of radio telescopes could record and process.
In 2007 a small precursor to the EHT took advantage of these trends and used a trio of telescopes in Hawaii, California and Arizona to pierce the veil surrounding Sagittarius A*. The result was far from an image, but the project saw something—presumably, light from the long-sought shadow.
The first prediction of black hole shadows came in 1973, when physicist James Bardeen showed that a black hole in front of a bright background would produce a silhouette. He decided that “there seems to be no hope of observing this effect.” In 2000, however, astrophysicists Heino Falcke, Fulvio Melia and Eric Agol demonstrated that a microwave-gathering, Earth-size radio telescope should be able to see the shadow of Sagittarius A*.
Half a decade afterward, a few dozen of the astronomers and astrophysicists laboring in this obscure corner of astronomy agreed on the formal goal of building a virtual planet-scale radio telescope to observe that shadow. The first official kickoff meeting for the project occurred in January 2012, and the Event Horizon Telescope was born.
Five years later, after growing into a collaboration of more than 200 scientists with eight participating observatories across the globe, the EHT took its first realistic shot at seeing the shadow of Sagittarius A*. Over the course of 10 days in April 2017, telescopes in North America, South America, Hawaii, Europe and Antarctica collectively zoomed in on the galactic center and other black holes, gathering 65 hours of data on 1,024 eight-terabyte hard drives, which were shipped to supercomputer banks in Massachusetts and Germany for correlation. Five years after that, the elated EHT researchers showed the world that their experiment had worked.
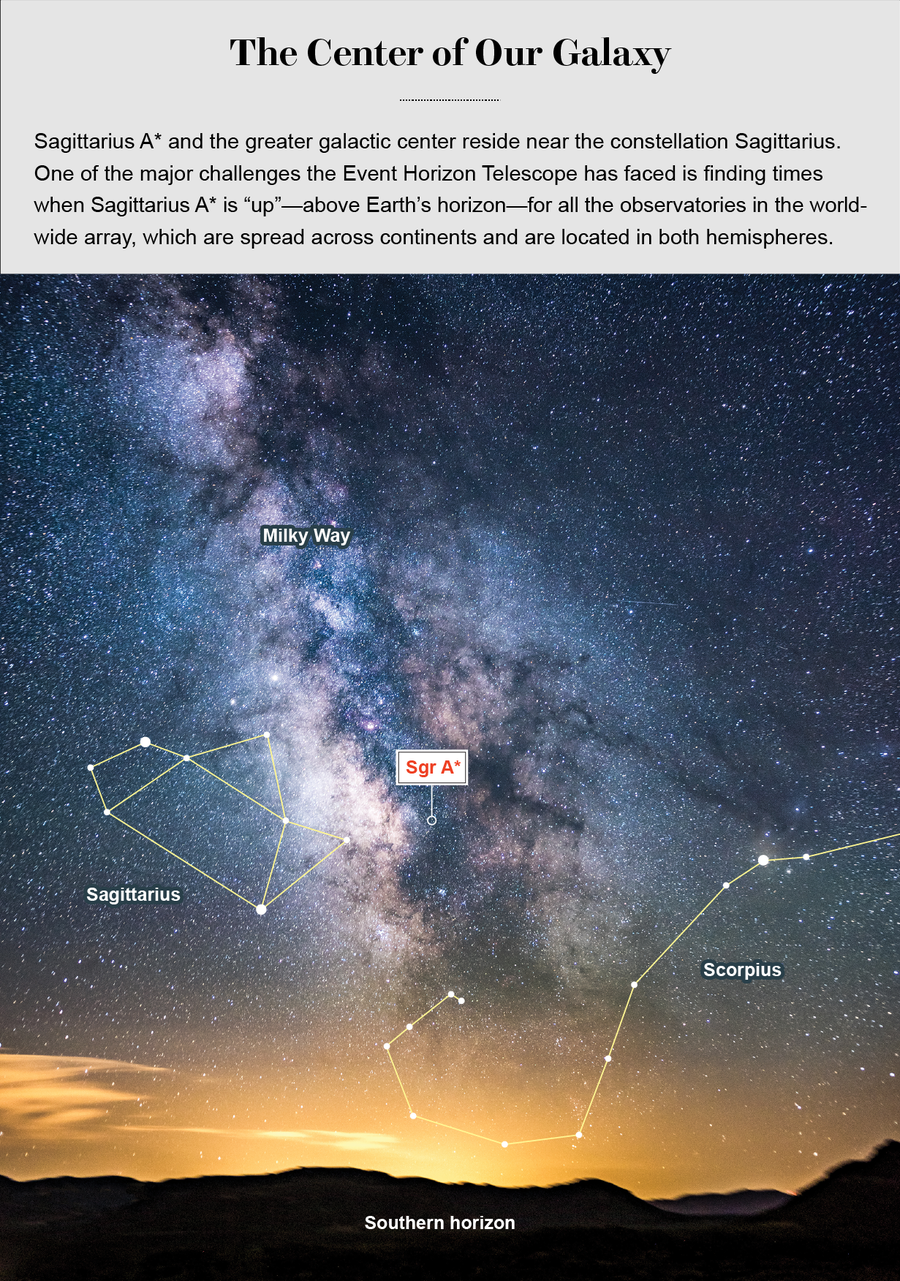
Katie Peek (graphic overlay); Eloi Omella/Getty Images (Milky Way photograph)
The day the EHT collaboration revealed Sagittarius A*’s image, the Astrophysical Journal Letters published a special issue devoted to the new results. In six technical papers, the scientists presented a multidimensional portrait of our black hole.
The EHT image confirmed the basics. We’ve long known that Sagittarius A* is about 27,000 light-years away. Years spent tracking the orbits of stars around Sagittarius A* with infrared telescopes had already given astronomers an accurate measure of the black hole’s mass—the equivalent of roughly four million suns. Plug those two numbers (distance and mass) into equations derived from general relativity, and you can calculate the expected size of the black hole’s shadow. Sure enough, the image matches the prediction. The shadow has a diameter of 52 micro arc seconds, which means that to us here on Earth it is, in the astronomers’ formulation, the size of a “doughnut on the moon.” After seeing the shadows of both Sagittarius A* and M87*—black holes that differ in mass by three orders of magnitude—the scientists concluded that the phenomena are “universal features of black holes.”
The EHT observations, combined with simultaneous monitoring by the Chandra and Nu-STAR x-ray telescopes and other instruments, are starting to settle long-standing questions about Sagittarius A*’s environment. By measuring the spectra of light shining from the object—that is, the light broken up into its constituent frequencies—astronomers long ago determined that the matter orbiting the black hole is a diffuse gas of electrons and protons. We now have a much better idea of where that matter comes from. Observations from the Chandra x-ray telescope show that the black hole pulls that matter from the atmospheres of stars orbiting it. Not that it pulls very much. Sagittarius A* is on a starvation diet—less than 1 percent of the stuff captured by the black hole’s gravity ever makes it to the event horizon. That explains why the black hole is so dim. Despite being four million times more massive than our sun, Sagittarius A* is only 100 times brighter.
It wasn’t always so faint. It appears that as recently as 60 or 70 years ago, Sagittarius A* went on a feeding binge, a burst of activity that left “light echoes”—light bouncing off nearby clouds of dust and gas—that astronomers have detected with x-ray telescopes. And it still has its active moments. On April 11, 2017, Sagittarius A* emitted a bright x-ray flare. The cause, most likely, was the twisting and recombining of magnetic fields inside the matter orbiting the black hole—the same basic dynamic that causes solar flares in our own star. Directly observing these flares is a major goal for future campaigns.
There’s much more to learn about Sagittarius A*. For example, the EHT observations show that, like everything else in space, the black hole is spinning—but we don’t know how fast. Future observations also aim to show exactly how the black hole eats infalling matter and, with luck, make movies of the black hole evolving over time.
The first EHT picture of Sagittarius A* is just the beginning, but it does tell us what the object is not. The presence of a distinctive shadow means Sagittarius A* has an event horizon—the defining feature of a black hole. That means we finally know it’s not a really, really, really dense star, or a wormhole, or a naked singularity (a point of infinite density unconcealed by an event horizon), or any of the other exotic oddities theorists have proposed over the years. It’s nothing stranger than a supermassive black hole—still a pretty strange thing indeed—now brought within view, closer to revealing its secrets.